Aside from mechanical codes that mandate certain safety details, there is virtually no limit to how a hydronic heating system can be assembled.
After three decades of working with this extreme flexibility, I see it as both a blessing and a curse. A blessing in that creative designers, with the right experience and design tools, can customize a hydronic system to the exact needs of a building and its occupants. And a curse because people lacking these resources can quickly assemble thousands of dollars worth of hardware into an aberration that causes frustration, wastes fuel, creates possible safety issues and spawns another bad rap for hydronic heating.
Blame game
On several occasions I’ve listened as building owners, their attorneys, the architects or even the installers blame the hydronic system’s boiler as the source of inadequate heating. Their accusations range from “The boiler obviously isn’t big enough” to “There must be something wrong with the burner” to “My big old boiler always heated the place fine.” This is like naively blaming the engine for every malady the ever besets an automobile.Here’s a question I like to ask after hearing such accusations: “When it’s very cold outside, does the boiler run almost all the time?” With few exceptions the answer is no.
What might you conclude from this response? The likely explanation is that the boiler is reaching its high limit setting and shutting off because it’s creating heat faster than the remainder of the system can dissipate that heat. In other words, one or more “bottlenecks” exist in the heat transfer process somewhere between the boiler and the heat emitters. Although I don’t have statistical evidence to prove it, my qualitative assessment is that such bottlenecks, rather than the boiler, are responsible for most complaints involving inadequate heat delivery.
Hydronic bottlenecks fall into two categories: flow bottlenecks and heat transfer bottlenecks. Both can lead to complaints of inadequate heat output. This month we’ll look at some common flow bottlenecks.
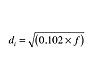
Formula
1
It's not the boiler's fault
A boiler that is truly undersized for the load is a possibility, but in my experience it’s also rare. Most boilers are generously oversized for the load, especially in cases where they have been selected without reference to an accurate heating load calculation.Still, it doesn’t really matter how much extra capacity the boiler has if the “conveyor belt” - the distribution system and heat emitters - between it and the spaces being heated can’t do its job.
Following are some common flow bottlenecks and solutions.
Formula 1 can be used to find the minimum internal diameter of a pipe based on the flow rate it must carry while not exceeding a flow velocity of 4 ft. per second.
Formula 1

di = minimum internal diameter of pipe (inches)
f = flow rate (gpm)
Find the minimum internal diameter of a pipe to carry a flow of 15 gpm, such that the flow velocity doesn’t exceed 4 ft. per second:
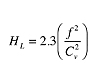
Formula
2
One of the classics is when a typical 80 watt wet rotor zone circulator is installed to create flow between a relatively large (200,000+ Btu/hr.) boiler and a 119-gallon indirect water heater. To move 200,000 Btu/hr. based on a circuit temperature drop of 20 ºF requires a flow of 20 gpm. This is well above what a small zone circulator can handle. The situation is only exasperated when 3/4-in. tubing is used for the circuit.
I think this happens because some installers see the indirect water heater as just another heating zone, and thus it can have the same circulator as the other zones. However, when the indirect operates as a priority load, the circulator and piping should be sized to deliver all the heat output of the boiler to the tank.
The greater the total equivalent length of a circuit, the lower the flow rate produced by a given circulator. So play close attention to how equivalent lengths add up if you are creating series circuits.
Three-way thermostatic mixing valves for tempering domestic hot water were never designed for mixing in typical hydronic heating systems. These valves have relatively small internal ports that add considerable flow resistance and pressure drop at higher flow rates.
Most 3/4-in. and 1-in. sizes of such valves have Cv ratings in the range of 2.5 to 4. The Cv rating is the flow rate through the fully open device, in gallons per minute, that creates a 1 psi pressure drop across the device. To put it in other terms, a valve with a Cv of 3.0 has an equivalent length of about 100 ft. of 3/4-in. tubing. So instead of that little brass valve, picture an extra 100 ft. of tubing in your circuit.
Formula 2 can be used to estimate the head loss of a device with a given Cv at other flow rates:
Formula 2
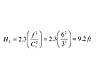
HL = head loss (feet of head)
f = flow rate (gpm)
Cv = Cv value of the device (fully open)
Suppose that a 3/4-in. thermostatic mixing valve with a Cv of 3.0 is installed to supply water to an eight-circuit radiant panel manifold station. The anticipated flow in each circuit is 0.75 gpm. The head loss through the thermostatic valve would be as follows:
This is a substantial portion of the head added by a typical zone circulator operating at 6 gpm, and it’s only the head loss of the valve. The remaining piping and the radiant panel circuits will likely create significantly more head loss. Too much head loss = too little flow. Too little flow = too little heat transport. Too little heat transport = too many complaints.
Can you overcome this higher head loss with raw pumping power? Sure, but it’s going to require a circulator that costs quite a bit more and has a much larger appetite for kilowatt hours. It’s always better to reduce head loss rather than upsize the circulator.
The industry has since learned that lesson, and now does a good job in showing how to pipe a high-flow resistance boiler as a separate circuit that’s hydraulically separated from the remainder of the distribution system. But some people don’t follow instructions. Instead, they simply reconnect the supply and return piping from an existing distribution system to a new compact boiler. The result is an instant flow bottleneck that will substantially reduce zone flow and heat delivery. Inevitably the new boiler gets the blame, regardless of what the I/O manual specifies for proper installation.
Using them for component isolation is like driving your car around, year after year, with the parking brake partially applied. Over the life of a hydronic system, these valves spend tens of thousands of hours dissipating unnecessarily higher amounts of head energy from the flowing water.
As you design hydronic systems, especially those expected to handle large loads, keep your eyes open for any components or piping assemblies that might impede flow. If you suspect a potential problem, do the calculations to assess the impact on flow. Then decide if the component or assembly is acceptable, or if a different approach is needed to avoid a flow bottleneck.
Remember that every hydronic circuit you will ever design will always settle to a flow rate where “hydraulic equilibrium” exists between the head added by the circulator and the head dissipated by fluid friction through the circuit. Be sure you select equipment so this flow can carry the required heat along for the ride.