Beware of bottlenecks, Part 2
Sometimes the Btu just canít squeeze through.
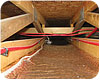
Last month we discussed several flow bottlenecks and how they can hinder system performance. This month we’ll look into some thermal bottlenecks: Situations in which something creates an excessive obstruction along the path from where heat is produced to where it’s supposed to be delivered.
Think about the contact (or lack thereof) between the tubing and the surrounding materials. Without good contact, conduction heat transfer is severely restricted. Although some heat transfer will be by convection and radiation, both of these effects are relatively weak, given the temperatures and geometry involved.
Without good conduction, the surrounding materials are unable to “wick” heat away from the tubing and spread it across the panel surface at a rate that keeps pace with the room’s heat loss. Under such conditions, the first law of thermodynamics mandates a decrease in room temperature until a balance between heat input and heat loss is established. Given the installation shown in Figure 1, I can assure you this balance will be well below desired comfort conditions.
The temperature drop of the water flowing through the circuit will be very low. This implies that most of the heat loaded on at the heat source just made the round trip without jumping off. There may be plenty of heat in the water, but it just can’t get out based on the poor thermal bridge between the tubing and remainder of the panel. My recommendation has remained the same for years: Stay away from plateless staple-up radiant floor heating.

Imagine this tank connected to a multiple boiler system capable of 400,000 Btu/hr. heat output, as shown in Figure 2.
What’s going to happen in this system upon a call for prioritized DHW heating? Here’s the likely sequence: The boiler staging control understands it has a call for DHW heating and instantly targets a high water supply temperature such as 190°. The staging control then fires up both boilers in short order to quickly drive the water supply temperature toward its target value. This is especially true if the boilers have been off for a while and thus are quite a bit cooler than the target supply temperature.
However, since the heat exchanger in the indirect tank can’t accept heat into the potable water as fast as the boilers are now producing it, the boiler temperature climbs rapidly. Remember, thermodynamics wants a balance between heat input and heat dissipation and will drive the water temperature up or down to achieve this balance whenever necessary. It won’t be long before the boiler staging control shuts off one or both boilers because the water temperature quickly reaches the target value and would likely overshoot if the boilers remained on any longer.
The staging control doesn’t understand a thermal bottleneck to heat transfer exists in the heat exchanger. Even if it did, there’s nothing it could do to correct the situation. Obviously a boiler that’s off, even for a few minutes, is not producing heat to help meet a high DHW demand. The tank’s heat exchanger, and not boiler capacity, is the thermal bottleneck limiting how fast DHW can be supplied.
There are several possible ways to avoid this situation. One is to select an indirect tank with an internal heat exchanger capable of accepting the full output of the boiler (or multiple boiler system) without exceeding a boiler supply temperature of 180°. If you really want to maximize efficiency, you might even want to limit the boiler outlet temperature to a lower value such as 150° to 160°. Just be sure the tank’s heat exchanger can handle the total output of the boiler system at this supply temperature.
Another possibility is using an external stainless-steel, brazed-plate heat exchanger sized using the same criteria as just discussed. This will require an extra circulator compared to the indirect tank approach. That circulator moves potable water between the storage tank and heat exchanger, so it has to be either stainless steel or bronze.
One distinct advantage of this approach is the external heat exchanger can always be cleaned, or replaced if ever necessary, without removing the storage tank. Another advantage is the wide variety of brazed-plate heat exchangers available to handle almost any heat transfer requirement in a residential or light commercial application.
Yet another possibility is to install an additional storage tank and thus increase the available heat exchanger area between the boiler water and potable water. This would not be my preferred solution. The cost of installing two indirect tanks is likely to be more than that of an external heat exchanger, stainless-steel circulator and standard storage tank. Two tanks also will require more space in the mechanical room.
Most importantly, the extra tank significantly adds to tank surface area, which increases standby heat loss. This extra heat loss is likely to be the big expense over the life of the system, especially when you consider how it adds to the building’s cooling load in warm weather. It’s always better to store energy in a high-grade form (e.g., fuel) rather than converting it to a lower-grade form (heat) until just before the lower-grade energy is needed.
In retrofit projects, it is common to find standard fin-tube baseboard or radiators sized around much higher supply temperatures. Such heat emitters simply can’t deliver the necessary heat output when operating at significantly lower design water temperatures.
If you’re involved with retrofitting an existing hydronic distribution system to a low-temperature heat source, it’s imperative to do an “inventory” of the existing heat emitters. I recommend doing this on a room-by-room basis. While you’re at it, do a room-by-room heat loss estimate based on the current thermal envelope of each room. Don’t assume that the existing heat emitters are properly sized for the current load.
Next, calculate the heat output from the existing heat emitter(s) based on the lower design water temperature. Compare this heat output to the design heating load; from there determine what (if any) supplemental heat input is required.
The versatility of hydronics is your friend in such circumstances. You may be able to add more standard baseboard, go to high-output fin-tube baseboard, incorporate a small area of radiant wall or ceiling heating, or add a small fan coil. The goal is to add enough heat emitter surface area to allow all room loads to be met by a design supply water temperature no higher than 120°.
As you design hydronic systems, especially those expected to handle large loads, keep your eyes open for any bottlenecks that might constrict flow or heat transfer. Be sure the full output of the heat source(s) can reach the intended loads and be released by the heat emitters.
In every system, the temperatures and flow rates will settle to values where a balance occurs between energy production and energy dissipation. Be sure you select equipment so this balanced condition also provides the expected comfort levels in the building.
For Part 1, read here.
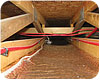
Figure 1.
Last month we discussed several flow bottlenecks and how they can hinder system performance. This month we’ll look into some thermal bottlenecks: Situations in which something creates an excessive obstruction along the path from where heat is produced to where it’s supposed to be delivered.
Thermal constipation
One of the classic thermal bottlenecks in modern hydronics is when the tubing in a radiant panel is “poorly connected” from the standpoint of heat transfer. A plateless staple-up installation, such as shown in Figure 1 below, is a common example.Think about the contact (or lack thereof) between the tubing and the surrounding materials. Without good contact, conduction heat transfer is severely restricted. Although some heat transfer will be by convection and radiation, both of these effects are relatively weak, given the temperatures and geometry involved.
Without good conduction, the surrounding materials are unable to “wick” heat away from the tubing and spread it across the panel surface at a rate that keeps pace with the room’s heat loss. Under such conditions, the first law of thermodynamics mandates a decrease in room temperature until a balance between heat input and heat loss is established. Given the installation shown in Figure 1, I can assure you this balance will be well below desired comfort conditions.
The temperature drop of the water flowing through the circuit will be very low. This implies that most of the heat loaded on at the heat source just made the round trip without jumping off. There may be plenty of heat in the water, but it just can’t get out based on the poor thermal bridge between the tubing and remainder of the panel. My recommendation has remained the same for years: Stay away from plateless staple-up radiant floor heating.

Figure 2.
DHW asphyxiation
Indirect water heaters that supply high-demand DHW loads also can create thermal bottlenecks. For example, a large 119-gallon indirect water heater may have a coil rating in the range of 250,000 Btu/hr. when supplied with boiler water in the range of 180° to 190° F and a flow rate of about 26 gpm.Imagine this tank connected to a multiple boiler system capable of 400,000 Btu/hr. heat output, as shown in Figure 2.
What’s going to happen in this system upon a call for prioritized DHW heating? Here’s the likely sequence: The boiler staging control understands it has a call for DHW heating and instantly targets a high water supply temperature such as 190°. The staging control then fires up both boilers in short order to quickly drive the water supply temperature toward its target value. This is especially true if the boilers have been off for a while and thus are quite a bit cooler than the target supply temperature.
However, since the heat exchanger in the indirect tank can’t accept heat into the potable water as fast as the boilers are now producing it, the boiler temperature climbs rapidly. Remember, thermodynamics wants a balance between heat input and heat dissipation and will drive the water temperature up or down to achieve this balance whenever necessary. It won’t be long before the boiler staging control shuts off one or both boilers because the water temperature quickly reaches the target value and would likely overshoot if the boilers remained on any longer.
The staging control doesn’t understand a thermal bottleneck to heat transfer exists in the heat exchanger. Even if it did, there’s nothing it could do to correct the situation. Obviously a boiler that’s off, even for a few minutes, is not producing heat to help meet a high DHW demand. The tank’s heat exchanger, and not boiler capacity, is the thermal bottleneck limiting how fast DHW can be supplied.
There are several possible ways to avoid this situation. One is to select an indirect tank with an internal heat exchanger capable of accepting the full output of the boiler (or multiple boiler system) without exceeding a boiler supply temperature of 180°. If you really want to maximize efficiency, you might even want to limit the boiler outlet temperature to a lower value such as 150° to 160°. Just be sure the tank’s heat exchanger can handle the total output of the boiler system at this supply temperature.
Another possibility is using an external stainless-steel, brazed-plate heat exchanger sized using the same criteria as just discussed. This will require an extra circulator compared to the indirect tank approach. That circulator moves potable water between the storage tank and heat exchanger, so it has to be either stainless steel or bronze.
One distinct advantage of this approach is the external heat exchanger can always be cleaned, or replaced if ever necessary, without removing the storage tank. Another advantage is the wide variety of brazed-plate heat exchangers available to handle almost any heat transfer requirement in a residential or light commercial application.
Yet another possibility is to install an additional storage tank and thus increase the available heat exchanger area between the boiler water and potable water. This would not be my preferred solution. The cost of installing two indirect tanks is likely to be more than that of an external heat exchanger, stainless-steel circulator and standard storage tank. Two tanks also will require more space in the mechanical room.
Most importantly, the extra tank significantly adds to tank surface area, which increases standby heat loss. This extra heat loss is likely to be the big expense over the life of the system, especially when you consider how it adds to the building’s cooling load in warm weather. It’s always better to store energy in a high-grade form (e.g., fuel) rather than converting it to a lower-grade form (heat) until just before the lower-grade energy is needed.
Undersized heat emitters
Increased use of renewable heat sources such as solar collectors, geothermal water-to-water heat pumps and air-to-water heat pumps is driving the hydronics industry toward low-temperature distribution systems. We discussed this trend in several recent columns. It’s happening in North America as well as globally. These systems must include heat emitters that can operate at low water temperatures. My recommendation is that any distribution system supplied by a renewable heat source should be able to deliver full design-day heat output while operating at supply water temperatures no higher than 120°.In retrofit projects, it is common to find standard fin-tube baseboard or radiators sized around much higher supply temperatures. Such heat emitters simply can’t deliver the necessary heat output when operating at significantly lower design water temperatures.
If you’re involved with retrofitting an existing hydronic distribution system to a low-temperature heat source, it’s imperative to do an “inventory” of the existing heat emitters. I recommend doing this on a room-by-room basis. While you’re at it, do a room-by-room heat loss estimate based on the current thermal envelope of each room. Don’t assume that the existing heat emitters are properly sized for the current load.
Next, calculate the heat output from the existing heat emitter(s) based on the lower design water temperature. Compare this heat output to the design heating load; from there determine what (if any) supplemental heat input is required.
The versatility of hydronics is your friend in such circumstances. You may be able to add more standard baseboard, go to high-output fin-tube baseboard, incorporate a small area of radiant wall or ceiling heating, or add a small fan coil. The goal is to add enough heat emitter surface area to allow all room loads to be met by a design supply water temperature no higher than 120°.
As you design hydronic systems, especially those expected to handle large loads, keep your eyes open for any bottlenecks that might constrict flow or heat transfer. Be sure the full output of the heat source(s) can reach the intended loads and be released by the heat emitters.
In every system, the temperatures and flow rates will settle to values where a balance occurs between energy production and energy dissipation. Be sure you select equipment so this balanced condition also provides the expected comfort levels in the building.
For Part 1, read here.
Links
Looking for a reprint of this article?
From high-res PDFs to custom plaques, order your copy today!