Microflows
John Siegenthaler
I’m excited about what’s in store for hydronic heating in 2007. For one thing, the North American market will be getting its first real encounter with state-of-the-art “smart circulators.” These little devices, now being installed by the thousands in Europe, can glean significantly more head out of every watt of input power. In my opinion, they represent a milestone in the history of hydronic system technology, and are every bit as significant as recent advances in small modulating/condensing boilers.
The energy appetites of these new circulators stand in stark contrast to the wattage demand of wall-to-wall racks of wet rotor circulators often found in North American residential heating systems. Although these over-pumped systems work, they consume far more electrical energy than European systems of equivalent thermal capacity.
Now that we’ve just about hit the ceiling for combustion efficiency, it’s time for our industry to pay more attention to electrical energy conservation in the hydronic systems we design. We should take it as a challenge to see what’s possible before assuming a circulator is needed on every zone. That’s what this month’s column is about, and the findings may surprise many of you.

Flashback
I grew up in a house where a single B&G Series 100 circulator supplied a split loop baseboard system. Although it was far less sophisticated than many currently installed systems, I remember that just one circulator handled the entire house and handled it well.Fast-forward to 2007. Is it still possible to design a single circulator system for a typical suburban house and retain the benefits of room-by-room zoning, the thermal efficiency of mod/com boilers, and the installation convenience of modern piping materials?
I can’t ponder these things very long without throwing some numbers into the picture to see if concept can make it to reality. So here we go…
Imagine a typical 2,500-square-foot North American house with a design heating load of 25 Btu/hr./square foot (total heating load of 62,500 Btu/hr.). Such a house would certainly not qualify as superinsulated. Instead, it would be near the lower fringes of what some state energy codes currently tolerate. Think of it as a builder’s “spec house” in a typical suburban development.
The hydronic heating system I selected for this example house uses panel radiators and a homerun distribution system. I did this for several reasons:
- 1. Panel radiators come in a variety of heights, widths and depths allowing them to fit a wide variety of situations.
2. Their thermal performance is not affected by floor coverings, and hence they’re not as limited as floor heating might be in some situations.
3. They’re one of the easiest heat emitters to install in new homes as well as in retrofit applications. Because they’re relatively light, they can be supported by almost any wall.
4. They lend themselves to room-by-room zoning using nonelectric thermostatic radiator valves. This provides fully modulated room-by-room comfort control without a mile of thermostat cable and a box full of wire nuts.
5. Because people don’t walk on panel radiators, they can operate with a higher temperature drop than residential floor heating circuits. The latter are expected to provide barefoot-friendly floors and hence are limited to temperature drops of 15 to 20 degrees F.
A schematic of the proposed system is shown in Figure 1.
A simple copper manifold system supplies eight panel radiators, each on its own homerun circuit. Manifold flow is provided by a single fixed-speed circulator. Heated water is provided from a small 80,000 Btu/hr. modulating/condensing boiler connected to a well-insulated 15-30 gallon buffer tank. The latter was included to reduce boiler cycling under the very low “microload” conditions possible given the extensive zoning of this system. Domestic hot water is provided by an indirect water heater operated as a priority load.
Although there are boilers available with lower heat outputs, I prefer to keep the 80,000 Btu/hr. model in the system to provide reasonable recovery rates for domestic water heating.
For simplicity, I assumed each panel radiator handles a design load of 8,000 Btu/hr. I also assumed the water temperature supplied to the panels at design conditions will be 160 degrees F with a temperature drop of 40 degrees F. This relatively high temperature drop is a key factor in reducing flow and pumping requirements while still allowing the heat emitter to meet load without compromising comfort.
The radiators I selected for this system are DiaNorm Model 22 panels with a height of 24 inches and length of 72 inches. These panels release about 8,400 Btu/hr. each when operating at an average water temperature of 140 degrees F in a room at 68 degrees F.
The flow rate required for each panel at design load would be (Formula 1):

Formula 1
Where:f = flow rate (in gpm)
Q = rate of heat transfer (Btu/hr.)
490 = a constant based on water as the system fluid
∆T = temperature drop across the panel (degrees F)
This flow rate could easily be handled by 3/8-inch tubing.
Simulated Reality
I used the Hydronics Design Studio software to create the homerun circuits and see what circulators would be up to the task. Doing so requires specified lengths of tubing as well as head loss information for the panel radiator.Based on manufacturer’s data, the head loss of the panel radiator is essentially that of the valve body mounted in its upper right corner. The multiple parallel risers in the two wetted faces of the radiator reduce dynamic head loss of the panel itself (excluding the valve) to almost zero. The head loss of the panels selected can be calculated using Formula 2, which assumes the integral radiator valve is fully open.
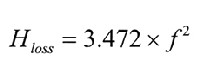
Formula 2
Where:Hloss = head loss of panel (feet of head)
f = flow rate (in gpm)
Based on this head loss, it’s possible to calculate the equivalent length of the panel. This is simply that length of a specific type and size tubing that has the same head loss as the radiator. For more information on calculating equivalent length, see the June 2005 Hydronics Workshop column entitled “Equivalent Thinking.” It’s available online in the PM archives (free site registration required).
In this case, each radiator has the following calculated equivalent lengths:
- Equivalent length of radiator for 3/8-inch PEX tubing = 21 feet
- Equivalent length of radiator for 1/2-inch PEX-AL-PEX tubing = 100 feet
Using a Grundfos UPS15-58 on speed 1 (60 watts rated power consumption), each panel receives a flow of 0.52 gallons per minute, more than sufficient for the required performance (see Figure 2). This means that a single 60-watt circulator is more the capable of handling design loading, even when supplying the panels through 3/8-inch PEX tubing. That’s right: 60 watts can push water through tubing smaller than your pinky and heat an entire 2,500-square-foot, moderately insulated house on a design day.

It Gets Better
I use the term “distribution efficiency” to measure how well a heating system delivers the heat produced in the mechanical room to the heat emitters. Mathematically, it’s simply the rate of heat delivery divided by the wattage used to make that delivery.The distribution efficiency of the single circulator system described would be (Formula 3):

Formula 3
Here’s something to compare that number to: A forced-air system supplied by a heat pump of equivalent capacity has a distribution efficiency of about 56 Btu/hr./watt. Thus, the hydronic system delivers fully zoned superior comfort using only about 5 percent of the electrical wattage of the forced-air distribution system. Does anybody see a sellable benefit here?The next simulation used the same distribution system coupled to a B&G NFR-9F/LW circulator (40 watts rated power consumption). The flow through each panel was 0.44 gpm, almost exactly the same as the target flow rate calculated for the 40 degree F design temperature drop. In this case, distribution efficiency jumps up to 1,680 Btu/hr./watt, 30 times higher than the forced-air heat pump system.
Similar results are obtained with other currently available wet-rotor circulators with motors rated from 40 to 60 watts.
Imagine a single circulator - perhaps one designed for DHW recirculation - handling the entire heat distribution within a house that might otherwise be equipped with a 5-ton-rated geothermal heat pump. Such a heat pump would have a nominal 1-horsepower PSC blower motor drawing 1,200+ watts to push air through the duct system.
It would also require an additional 400 watts of pumping power to circulate fluid through a closed geothermal piping loop. The life-cycle operating cost for heat collection and distribution in this geothermal heat pump system would be several thousand dollars more than the hydronic system described above. These numbers do help keep things in perspective, don’t they?
It’s also important to remember that both simulations were design load conditions where the system is capable of delivering about 67,000 Btu/hr. to the house. Under partial load conditions caused by temperature setbacks or internal heat gains, the flow requirements and associated wattages drop even lower.
In theory, pumping power varies with the cube of flow rate. Cutting system flow in half theoretically drops pump wattage to 1/8 its previous value. Even allowing for the fact that real circulators, especially small ones, don’t precisely follow the pump affinity laws, it’s still possible they could operate at wattages in the single digits. That’s probably less than the standby power drawn by all those “black block” transformers plugged in to a typical home office for devices like printers, disc drives, modems and routers.
Seeing Is Believing
I recently saw performance labels on two state-of-the-art, variable-speed ECM motor circulators now available from different manufacturers in Europe. One indicates an operating wattage range of 6 to 50 watts, the other 5.8 to 34 watts. These are both current production “smart pumps” capable of automatically varying speed to maintain a set differential pressure as zone flows vary. Both eliminate the need for differential bypass valves. Both are stock items in Europe, and both are headed across the pond in the very near future.The thermodynamic properties of water combined with a high temperature drop and parallel piping enable you to create hydronic distribution systems that consume a fraction of the wattage needed by forced-air systems. The electrical energy savings make this approach every bit as “green” as solar collectors and wind turbines. Get your head around the idea of low wattage distribution systems because they will play a vital role in the future of North American hydronics technology.
Looking for a reprint of this article?
From high-res PDFs to custom plaques, order your copy today!