Dashed Expectations
John Siegenthaler
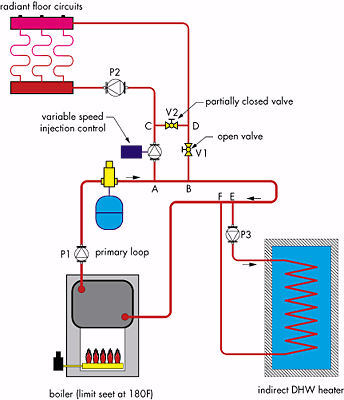
The piping schematic in figure 1 depicts one such case. The diagram arrived with a list of anomalies from its befuddled installer. The system was supposed to provide floor heating and year-round domestic hot water, a typical requirement for a new residential installation.
The progressively minded installer had selected primary/secondary piping as the backbone of the system. He also chose variable speed injection mixing to control water temperature in the radiant circuits. Confident that such modern techniques would surely deliver superior performance he piped away with great expectations, perhaps while thinking of submitting the project for an RPA System Showcase award.
After tightening the last flange bolt and twisting on the last wire nut he arrived at the moment of truth we all face on every job. A time when our pulse quickens in direct proportion to the complexity of the system that's about to come to life. That juncture where the master switch is flipped on and our design intentions are supposed to become reality. Will everything work as expected?
Such was not to be the case for this job. Soon after start-up its installer would wince in frustration as the pitiless physics of nature revealed the flaws in what moments earlier had been his proud accomplishment.
Some of the problems were immediately apparent as he moved his hand from one pipe to another feeling for the expected temperatures. The more latent deficiencies revealed themselves through repeated phone calls from a frustrated owner.
According to notes that came in with the schematic this system suffered the following anomalies:
- It allowed the water in the floor circuits to eventually reach the same temperature as the primary circuit (in this case 180 degrees F).
- It could not keep up with the domestic hot water demand during cold weather.
- Uncontrolled heat migration into the floor heating system, (probably more noticeable during mild weather).
- Higher than necessary stand-by heat losses from the DHW tank.
- Possible difficulty with purging or occasional air noises in the piping.
I'll bet many of you hydronic hawkeyes have already spotted one flaw in Figure 1. (Take a look at where the expansion tank is located relative to the primary loop circulator P1.) The circulator is pumping toward rather than away from the expansion tank. Not only does this make air removal harder than necessary, it also sets the stage for potential cavitation problems if the primary loop is operated at high temperatures and relatively low pressures. The fix is easy. Just relocate circulator (P1) so it pumps away from the expansion tank. If the frustrated installer adapted this detail on every future system he would soon find it hard to remember what "air problems" were like.

Injection Rejection
The piping details where the primary loop interfaces with the radiant floor system are loaded with errors. First of all, the tees at points A and B are too far apart. The pressure drop between them encourages hot water in the primary loop to flow up through the injection pump, even when it's off. The result is uncontrolled heat input to the radiant floor circuits anytime the primary loop is operating. In the dead of winter such thermal leakage may not be noticed. However, in milder weather it could easily lead to overheating.When the distribution circulator (P2) is running, the pressure drop created across the partially-closed valve (V2) further increases uncontrolled hot water flow to the radiant floor circuits. Although such a balancing valve is necessary when a two-way modulating valve is used to meter hot water into the radiant floor distribution circuit, it has absolutely no purpose in combination with a variable speed injection pump.
The fact that the injection risers goes up from the primary loop allows buoyancy-driven flow to carry hot water into the distribution system whenever there's flow in the primary loop. During warm weather, when the only thing the system should be heating is domestic water, such piping allows hot water to slowly but surely migrate through the floor heating circuits. It was only a matter of time until the complaint came in from the "overheated" owner.
Collectively, the above details make it impossible to control the rate of hot water being mixed into the radiant distribution loop. With piping like this, an otherwise accurate injection mixing controller operates about as well as a brain surgeon with a meat cleaver.
The piping schematic shown in Figure 2 corrects these problems. The tees at A and B, as well as at C and D are now spaced as close together as possible. The injection risers drop at least 18 inches from the tees in the primary loop to those in the radiant distribution loop. This creates the thermal trap necessary to resist hot water migration when the injection pump is off. The balancing valve (labeled as V2 in figure 1) has been removed. However, valve (V1) remains and should be partially closed to force the injection pump to run close to full speed under design heating load conditions. (See the December 2000 Hydronics Workshop column for ways to set this valve.)
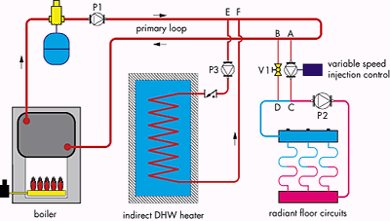
Domestic Deficiencies
Another shortcoming of the system was its inability to provide adequate domestic hot water during cold weather. Part of the problem is that the indirect water heater is piped downstream of the space-heating load. This reduces the water temperature available to the tank's heat exchanger when the radiant floor system is operating. The lower the water temperature supplied to the tank's heat exchanger, the slower it's recovery rate.Another problem is higher than necessary heat loss caused by gravity flow through the inactive secondary piping serving the tank's heat exchanger. Anytime a container of hot water has an unrestricted piping path that forms a loop from the top of the container to the bottom nature seizes the opportunity to use that path as a heat dissipater. In this case the loop includes the secondary piping supplying the tank's heat exchanger, and the short segment of primary piping between the tees labeled E and F in Figure 1.
There are three relatively simple changes that would significantly improve the performance of the DHW sub-system. One is to pipe the tank circuit as the first secondary circuit connected to the primary loop, and hence the recipient of the hottest water available in the system. The hotter the water entering the heat exchanger, the faster it can stuff Btus into the tank.
The second modification is to make the DHW circuit a priority load. Whenever the tank calls for heat the space heating sub-system is temporarily turned off, in this case by electrically removing the heat demand signal from the injection control. As soon as the tank has recovered, space heating is reenabled.
Last but not least, the off-cycle gravity flow problem can be corrected by installing a flow-check valve in the supply riser of the secondary circuit to the tank. It's weighted plug prevents flow through the tank's heat exchanger whenever circulator (P3) is off.
These modifications are shown in Figure 3.
Whenever an indirect water heater is piped in as a secondary circuit on a primary/secondary system the primary loop, as well as the DHW secondary piping, should be as short as possible and insulated to minimize heat loss in warm weather.
I think an even better approach is to pipe the DHW tank as a parallel circuit to the primary circuit. This eliminates the need to run the primary circulator during the DHW cycle. It reduces electrical use as well as piping heat loss. A flow-check valve must be installed in both the primary loop and the DHW piping loop when this arrangement is used.
Figure 4 shows the "made over" system with all of the above modifications. A flow-check valve has been added to the primary circuit downstream of circulator (P1). A swing check has been added where the primary loop returns to the boiler. These valves eliminate gravity flow through the primary loop when none of the loads are active. Purging valves have also been added to both secondary circuits to enable rapid air removal at start up.

Know Thy Details
The original layout used modern concepts like primary/secondary piping, indirect water heating, and variable speed injection mixing. However, without the proper detailing these state-of-the-art concepts failed to deliver the hoped for performance.High performance multiload hydronic systems seldom result from a "design-as-you-solder" approach. Instead they're crafted by consistently applying the basics. In this case understanding the effect of temperature drop along a series circuit, what conditions create gravity flow, and how to pipe in an injection pump. These basic details are then merged into one overall system respectful of all its parts.
Learn the basics, apply them consistently, and you'll install systems that perform as expected time after time.
Looking for a reprint of this article?
From high-res PDFs to custom plaques, order your copy today!