Picturing ∆P
John Siegenthaler, PE
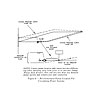
Just as heat flows from warm to cool, fluid flows from higher pressure to lower pressure. Pressure difference is the driving force for all flow in hydronic systems. It's definitely something worth understanding, if you plan to design properly performing hydronic systems.
Pressure difference is also the “evidence” that mechanical energy called head is being added or removed from a fluid as it flows through a hydronic system. An operating circulator adds head energy to the fluid. All other components through which the fluid flows remove head energy due to friction. The change in pressure is how we know this energy exchange is taking place. It's similar to a change in temperature indicating that thermal energy is being added or removed from a fluid.
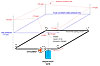
Can You See It?
One of my favorite drawings dealing with ∆P appears in a manual titled “Air Control for Hydronic Systems,” which was published in 1966 by Bell & Gossett. The drawing is shown in Figure 1a.Figure 1b shows a slightly embellished version of this drawing depicting the pressure at various points within a simple series piping circuit, when the circulator is off as well as when it's operating. It demonstrates the concept of PONPC (point of no pressure change), as well the difference between static and dynamic pressure.
The solid blue line “floating” above the piping circuit represents the static pressure in the piping directly below. Since all portions of the piping loop are shown at the same elevation, the static pressure is the same (let's assume 10 psi) at all locations. The height of the solid line above the piping represents the numeric value of static pressure in the loop. The higher the static pressure, the higher the line floats above the piping.
The dashed red line shown above the solid line represents the dynamic pressure in the piping when the circulator is operating. The word “dynamic” implies fluid motion is occurring.
Notice that the dashed line pitches up steeply as it passes over the operating circulator. This indicates a significant pressure increase between the circulator inlet and outlet. Again, this is evidence that head energy has been added to the fluid as it passed through the circulator.
As the dashed line proceeds clockwise around the circuit it slopes downward indicating head loss (e.g., mechanical energy being dissipated due to fluid friction). For simplicity the drawing shows a steady downward slope in the line implying that the piping is the same type and size at all locations.
The entire height gain of the dashed line across the circulator is “used up” by the downward sloping line as it passes around the remainder of the circuit. This is simply a visual way of indicating that the pressure gain across the circulator always equals the pressure drop around the circuit.

Lining Them Up
Figure 2 shows another way of visualizing ∆P in a circuit. This time it's the DHW circuit for a modulating/condensing boiler. In the lower part of the figure this portion of the system has been stretched into a line starting with the inlet of the circulator on the left and the tube that connects to the circulator inlet on the far right. For simplicity we'll assume that all components in this circuit are at the same height and thus that static pressure is equal in all areas of the circuit. The blue horizontal line represents this static pressure.The dashed red line shows the dynamic pressure when the circulator is running. Take a look at where the PONPC occurs. It's where the tube that “communicates” to the portion of the system containing the expansion tank connects to the DHW circuit.
Why is this the PONPC? Well, when the DHW circulator is running the check valve on the other (boiler) circulator is back-seated, and, thus, no flow passes through it. The only remaining connection between the DHW circuit and the expansion tank is along the pipe from point B to C in Figure 2. Hence, this connection becomes the pressure reference point for the DHW circuit relative to the expansion tank.
Given that both the left and right ends of the graph represent the same point (e.g. the circulator inlet) they must be at the same pressure. The pressures at the end of the sloping line segments in the dynamic pressure profile could be calculated knowing the specific flow rate and head loss characteristics of all the piping components in the circuits. The slopes shown are relative. They indicate a substantial pressure drop across the boiler's heat exchanger as well as across the DHW tank heat exchanger.
Notice how the dynamic pressure at several locations along the circuit drops below the static pressure when the circulator is operating. The lowest pressure occurs at the circulator inlet, and the highest pressure at its outlet. Although the circulator may still function properly under these conditions, it's generally not good practice to allow significant drops in pressure at the inlet of circulators. If the pressure at this location drops below the vapor pressure of the fluid cavitation will instantly occur.

Better Up Than Down
So what might be a better location for the tank? How about near the inlet of the DHW circulator as shown in Figure 3? Notice what happen to the dynamic pressure profile. The pressure at just about all locations along the DHW circuit increases because the PONPC is now very near the circulator inlet.Some of you may be wondering about the PONPC when the system operates in space heating mode. Keep in mind that the DHW circulator and boiler circulator do not run at the same time in this type of system. With the DHW circulator off the pressure reference for the space heating circuits is back through the DHW tank heat exchanger and on to the upper tee of the closely spaced tees upstream of the zone circulators. This puts the PONPC for the space heating mode very close to the inlet of those circulators, which is ideal.
Making a graph of dynamic pressure in a hydronic system can help in spotting problems such as excessive pressure drop in a given component, or circulator inlet pressures that may drop too low (possibly even sub-atmospheric). It's a good way of visualizing something we deal with every day in hydronic system design - ∆P.
Links
Looking for a reprint of this article?
From high-res PDFs to custom plaques, order your copy today!