Heating A 'Thermos Bottle' House
John Siegenthaler

The case supporting superinsulated house construction is getting another boost as fuel prices steadily increase. Construction techniques, such as structural insulated panels and insulated concrete forms, now make achieving these high R-value envelopes easier and faster than in the past. Although those who make their income installing heating systems may not admit it, it's often possible to show a better return on investment (ROI) for superinsulation upgrades to code-minimum thermal envelopes relative to spending equivalent funds on complex controls, heat sources or other hardware associated with a typical floor heating system.
Beyond ROI, there are other issues that detract from the use of heated floors in superinsulated houses. Chief among them is the fact that the floor will rarely feel warm. This happens because the rate of heat release from each square foot of floor is so low. Here's an example.
Consider a 2,000-sq.-ft. superinsulated home with a design heat loss of 18,000 Btu/hr. Assume that 90 percent of the floor area in this house is heated (e.g., 1,800 sq. ft.). The required upward heat flux from the floor at design conditions is:
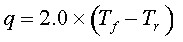

Tf = average floor surface temperature (degrees F)
Tr= room air temperature (degrees F)
q=heat flux (Btu/hr./ft.2)
To deliver 10 Btu/hr./ft.2, the floor only has to exceed the room temperature by 5 degrees F. Thus, for a room at 68 degrees F, the average floor surface temperature is only about 73 degrees F.
If you put your hand or bare foot on a surface at 73 degrees F, it will feel cool, about like placing it against an unheated interior partition. That's because your bare skin temperature is typically 80 to 84 degrees F. The house will be maintained at the desired indoor air temperature, but the cozy “warm floor” effect often anticipated by those desiring floor heating is simply not there. Bottom line: The owner is likely to be disappointed, especially if they probably paid a premium to get the warm floor effect so often touted in advertisements for radiant floor heating.
Hydronic Alternatives?
Some might argue that in the case of superinsulated houses, abandoning hydronic heat in favor of forced air is the answer. However, just because “full coverage” floor heating doesn't fit the situation doesn't mean other hydronic solutions aren't possible.
If your customer wants the “warm floor” effect in their superinsulated house, you must reduce the surface area of the radiant panel. Our industry's current approach to floor heating is to fill all available floor surface with tubing. This comes from wanting maximum output capability in houses with lots of windows or other details that tend to drive up heat loads. Distributing heat output over maximum surface area also lowers the system's operating temperature, which improves the efficiency of modern condensing boilers.
It's also hard to argue that such an approach doesn't increase sales of tubing and related hardware.
It's not always necessary or even desirable to treat the entire floor as a radiant panel. Reducing the heated area of the floor by half doubles the required upward heat flux. This in turn doubles the temperature difference between the floor surface and room air.
For example, instead of operating the entire floor area at a design flux of 10 Btu/hr./ft.2, you could heat half the floor area at a flux of 20 Btu/hr./ft2. This allows the average floor surface temperature to be about 10 degrees above the room temperature. Although still not likely to be considered “toasty,” this surface temperature probably will be perceived as comfortably warm.
You can use Formula 2, which is just a rearrangement of Formula 1, to estimate the average floor surface temperature based on a given rate of upward heat flux and room temperature.
Where:
Tf = average floor surface temperature (degrees F)
Tr= room air temperature (degrees F)
q=heat flux (Btu/hr./ft.2)
If you're going to reduce the heated floor area, be sure to think carefully about where the tubing will go. Avoid locations such as closets, under cabinets or areas where the floor is likely to be covered with furniture or area rugs. Favor locations like bathroom and kitchen floors, and floor areas adjacent to larger windows or doors. You might even consider “staging” some circuits in less critical areas so they only operate on the second stage of a thermostat.
Radiant walls and ceilings are another good option. Consider wall heating from just above the baseboard to the windowsill or chair rail height. Think about a 4-ft.-wide heated strip at the outer edge of the ceiling rather than all across the entire ceiling. Both of these approaches have very low thermal mass, allowing rapid response to internal heat gains. Both also will provide interior surfaces that are slightly warmer than those provided by full-coverage floor heating.
My favorite hydronic solution for low-energy-use houses is a panel radiator system using thermostatic radiator valves and a home-run distribution system. It can be supplied by one of the smallest modulating/condensing boilers along with a buffer tank. The concept is shown in Figure 1.
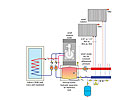
This approach also gives you the advantage of room-by-room temperature control with very low-mass heat emitters. When internal heat gains occur, the heat output from such a panel can stop very quickly. Room-by-room zoning, especially with nonelectric thermostatic radiator valves, is considerably simpler and less expensive than equivalent zoning using a forced-air system.
Given the low heating loads, you can size the panel rads for relatively low water temperature (think water supply temperatures of 120 degrees F or less at design load). This keeps the condensate flowing from the modulating/condensing boiler and provides noticeably warm surfaces on the panels during cold weather.
The buffer tank serves multiple duties as the air separating device and hydraulic separator between the boiler circulator and variable-speed distribution circulator. It allows a trickle of heat flow to the panels without constantly short-cycling the boiler.
Finally, remember that almost every superinsulated house needs domestic hot water. Installing a forced-air system for heating means a separate heat source will be needed for DHW. In a hydronic system, this is easily handled with an indirect tank. Be sure the DHW tank is well-insulated and heat trapped to ensure minimal standby losses. Such losses are much more likely to cause serious overheating in portions of a superinsulated house.
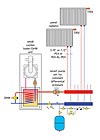
“Full coverage” radiant floorsystems may not be appropriate for every house. However, the wider spectrum of modern hydronic heating is almost infinitely adaptable to special-case situations. A carefully planned hydronic system can serve the heating needs of “micro-load” houses as well as a million Btu/hr. multiload system in private castle. In both cases, it's important to think beyond satisfying the thermostat to how the system will “feel” to the eventual occupants.
In the end, it's not just about efficiency and low energy use. Unrealized expectations, especially those involving personal comfort, do not lead to satisfied customers.
Links
Looking for a reprint of this article?
From high-res PDFs to custom plaques, order your copy today!