Seasons In The Sun
John Siegenthaler, PE
I entered the HVAC field in 1978 fresh out of college. My first job was as a junior engineer with Revere Solar & Architectural Products in Rome, N.Y. Our group manufactured flat plate solar energy collectors and designed both space heating and domestic water heating systems around them. Revere Solar was where I cut my teeth on hydronic system design. It also was where I began seeing the difference between wild theoretical heating concepts and what really worked in the field. It was an invaluable time of learning under the guidance of some very good mentors.
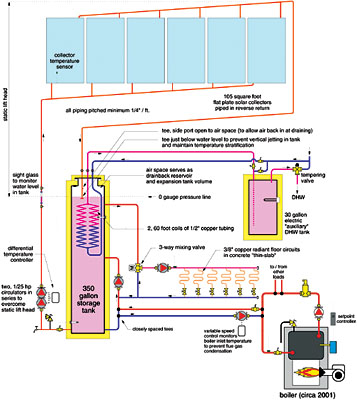
Practicing What I Preached
While employed at Revere, my wife Joyce and I also began building our home on evenings and weekends. As it took form, she envisioned a comfortable nest for our future family. I surreptitiously saw it as a live-in heating laboratory. After 24 years, I can honestly say it has served both purposes well.It probably doesn't surprise you that one of the systems I designed and installed in our house was an active solar energy system for supplying domestic hot water and some space heating. After considering many design options, I settled on the concept of a closed-loop gravity "drainback" system. This type of system uses a simple means of preventing water from freezing in the collectors and exposed piping. As soon as the collector pump turns off, gravity, a very reliable commodity, causes all water to drain from the collectors and pitched piping and back to a storage tank in heated space.
I liked the simplicity of the closed-loop drainback design, as well as the efficiency gains associated with not having a heat exchanger between the solar collectors and the storage tank water.
Figure 1 shows the present configuration of the system.
The solar portion (circa 1981) consists of six Revere flat plate collectors having a total area of 105 sq. ft. (see Figure 2). They supply a 10-ft. tall by 30-inch diameter vertical pressure-rated steel storage tank that was custom made for the system.
The tank's shape was chosen to encourage vertical temperature stratification. Cool water settles to the bottom of the tank while the hottest water rises to the top. A well-stratified tank can have a significant impact on solar system performance because collector efficiency is very dependent on inlet water temperature. The cooler the water, the higher the collector's efficiency, and the greater the amount of solar energy gathered.
Given the shape of this tank, one corner of the house was literally built around it. We actually hoisted and lowered the tank in place using a block and tackle, an old station wagon, and some (fortunately) strong roof trusses. Trust me: You would have been entertained by the show had you been there.
Operation of the drainback solar system is simple. A differential temperature controller monitors the temperature of collector absorber plate as well as the water near the bottom of the storage tank. When the absorber plate is at least 8 degrees warmer than the storage tank, the collector pump is turned on. When this temperature difference drops to 4 degrees or less, the pump is turned off.
In our system, the collector pump is actually a close-coupled assembly of two 1/25-horsepower circulators in series. This combination provides the ability to lift water from the static water level established in the tank to the top of the collector array. This system is not a fluid-filled piping loop as are the vast majority of "conventional" hydronic heating systems we discuss in other columns. The ability of the collector pump(s) to lift water and fill the collector array at the start of each collector operating cycle is critically important in this type of system.
Once the collector pumps are turned on, it take about 15 seconds for water to reach the top of the collectors, turn the corner at the upper elbow, and head back to the storage tank. The air in the collectors and exposed piping is pushed along by the entering water, and eventually returned to the top of the storage tank. The return pipe is sized so the flow velocity is at least 2 feet per second to entrain air bubbles and drag them back to the tank.
Inside the tank, the return pipe connects to two tees. One is installed a few inches above the static water level in the tank. It allows air to enter the return pipe when the collector pump shuts off, and thus lets the system drain. The other tee distributes the incoming flow horizontally across the upper portion of the tank to preserve temperature stratification. A sight glass was installed to monitor the water level in the tank to ensure the air inlet tee is never flooded.
The tank had a large 18-inch diameter flanged "personhole" at the top (in 1981, this detail was actually called a "manhole," but that's another story). The opening it provides allowed us to install two parallel-connected 60-ft. coils of 1/2-inch copper tubing for preheating domestic hot water. Piping the two coils in parallel greatly reduces pressure drop relative to a single 120-ft. coil.
The coil assembly was piped using dielectric unions to connections on the 1/2-inch steel top plate and lowered into the tank, right where the hottest water in the tank would surround it. The top plate was seated onto a silicone gasket and bolted in place, thus closing the system to the atmosphere.
In addition to providing a receiver volume for the drainback water, the air space at the top of the storage tank also serves as the system's expansion tank. We maintained the system under slight positive pressure just like any other closed-loop hydronic system. However, given the critical nature of the water level in the tank, we did not install any type of automatic fill valve. Very minor water losses from valve packings were replaced by adding water through a hose bib. If the tank needed a boost in pressure, it could be done using a small air compressor.
Eventually the silicone gasket lost its ability to maintain positive air pressure at the top of the tank. Although I could have pulled the tank cover and redone the seal, we found the system works just fine on the nominal 2 psi pressure created by the static water column in the tank. Given that the inside of the steel tank was coated with a cold galvanizing compound, I didn't feel a miniscule exchange of air would present any corrosion problems.

Expanding The Laboratory
When we built our house, the only backup to solar heating was a wood stove. It served its purpose well, but there came a time when we just didn't feel like stoking the flames every cloudy day. I also wanted to add floor heating in the garage, heat the outdoor pool in late spring, and continue the heating experiments in other ways. All signs pointed to adding a boiler to the system, which we did in 2001.The oil-fired boiler is connected to the storage tank using a variable-speed injection pump as the Btu bridge (see Figure 1). The sole function of the injection pump is to monitor the boiler inlet temperature and prevent the large thermal mass of the tank from pulling the boiler down into a sustained flue-gas condensing mode of operation.
The tank is maintained at about 120 degrees F by the boiler when the sun won't do the job. The DHW coil is thus constantly surrounded by hot water. The electric heating element in the 30-gallon "auxiliary" DHW tank very rarely comes on. The vast majority of the DHW load is supplied by either solar or oil-fired heat, rather than electricity.
The storage tank also makes a great buffer tank for the boiler. We routinely see burner on-cycles of one hour or more. The boiler operates at or close to steady state efficiency most of its operating time.
The one drawback of maintaining the storage tank temperature using the boiler is that it reduces the potential operating time of the solar collectors. However, given the cloud generator called Lake Ontario that lies 75 miles to our west, the amount of solar heat we pass up on in winter is really pretty small.
This Baby Really Cooks
Our family has lived with the solar portion of this system for 23 years. I did have a couple of tiny pinhole leaks in the absorber plates, which I soldered shut. Other than that, the system has worked with hardly a hiccup.What really has impressed me is the performance of the suspended copper tubing DHW heat exchanger. A single pass through this exchanger consistently brings the water very close to the temperature at the top of the tank. A three-way thermostatic tempering valve at the outlet of the auxiliary DHW tank is a must to prevent scalding water from passing to the faucets when the sun is out.
This system has produced tank temperatures of over 170 degrees F when several sunny days have occurred in sequence. It has also survived many bitterly cold nights with temperatures in the range of minus 30 degrees F. It provided the proof-of-concept installation I wanted before designing other gravity drainback systems. Its success paved the way for several other similar systems installed during the 1980s. Nearly all remain in operation today.
The experience of working with these systems has convinced me that a properly designed gravity drainback solar energy system is a solid performer. Next month we'll look at some ways to interface this solar subsystem with more contemporary hydronic components.
Looking for a reprint of this article?
From high-res PDFs to custom plaques, order your copy today!